Xerophytes
Some diagrams have Danish text. If it causes difficulties, the glossary at the end of the page may help.
What is a xerophyte?
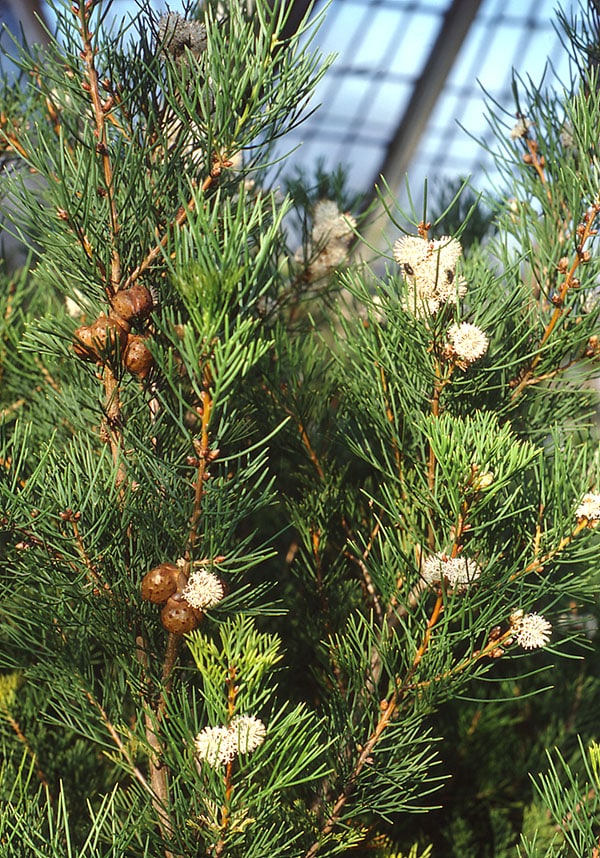
The term xerophyte originates from Greek and means a plant adapted to arid conditions. A xerophyte can grow where the precipitation is low, the soil dries out in short time, and air temperature is often high. These conditions occur e.g. in heath, dunes, semi-deserts, and deserts. Xerophytes can cope with these conditions because they have developed a number of morphological, anatomical, and physiological adaptations which reduce their transpiration and hence their water consumption and furthermore, many are able to store water in roots, stems or leaves for use in dry periods.
In the following, a number of different adaptations are discussed with the small Australian evergreen tree Hakea drupacea as an example. Then other examples follow from different countries. H. drupacea was originally named H. suaveolens, and I have published several papers under that name. It is not a distinct xerophyte, since it grows under relatively good precipitation in a coastal area along the southwestern and southern Australia, although in sandy well-drained soil and it should be able to survive a dry season. It is introduced in several places around the world, e.g. in South Africa and New Zealand. The picture to the left shows it flowering in a greenhouse in Copenhagen Botanical Garden.
When Hakea drupacea nevertheless possesses a large number of drought adapted (xeromorphic) characters, it is explained by the assumption that its ancestors were exposed to conditions making xeromorphic characters of great value, and since these characters in no way inhibit the species under the present conditions, the characters are not removed by natural selection.
The root system in Hakea is particularly effective in absor-bing water due to the development of so-called proteoid roots. It is a section of the roots with a vast number of short lateral roots, which greatly increase the absorptive surface of the root system as shown in the right Fig. (Lamont 1972).
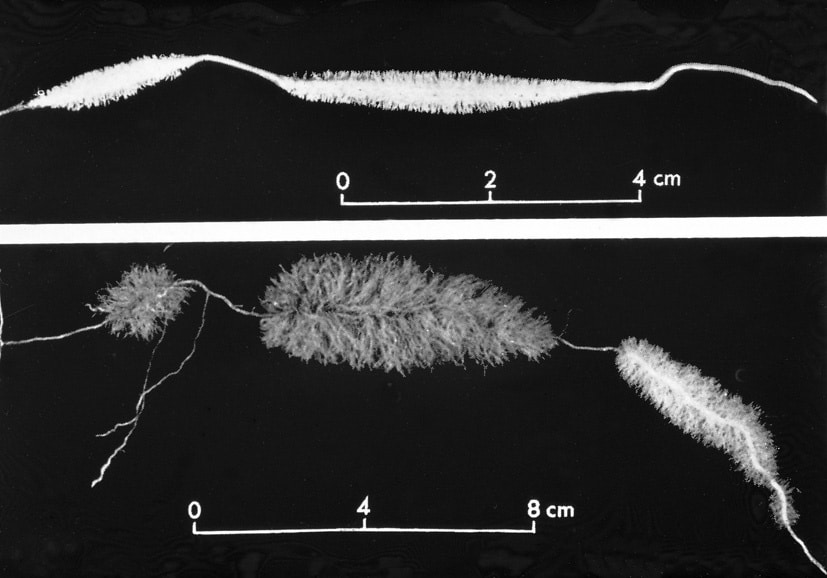
Generally, xerophytes have a deep and widely distributed root system but it may also be located near the soil surface to absorb rain, which only penetrates the uppermost 2-3 cm of the soil. As an example, the roots of a 25 cm high Opuntia cactus grow more than 125 cm to the sides. The diameter of the water conduction vessels in long roots is often extra-large to reduce diffusion resistance.
The needle-shaped cylindrical leaf or leaf segment is a xeromorphic character used by many species to save water and minimize evaporation from the leaf, i.e. reduce transpiration. At left, Hakea drupacea is shown together with a South-African xerophyte, Hymenolepis parviflora, having the same xeromorphic leaf shape. Conifers with needles are another example. Normally, conifers do not suffer from water stress in the growth season but when the soil in winter is frozen, they have the same problems with water supply as the xerophytes.
The Fig. above shows a sequence of Hakea drupacea leaves from cotyledon to mature leaf with cylindrical needle-shaped leaf segments. The bar is 2 cm. Hakea germinates at a time when the soil is not waterlogged and the seedlings will mostly be surrounded by protective vegetation. Therefore, it is only when the plant gets higher, meets drier air, and the leaves are warmed by the sun that there is a need to develop the xeromorphic leaf shape.
Transpiration increases when leaf temperature rises. Therefore, microphylli with small narrow leaves is an advantage for xerophytes. The two Figs. above illustrate along the red lines across a leaf with closed stomata how much leaf temperature can increase relative to ambient temperature. If the red-coloured areas farthest from the vascular system are removed on the broad leaf, as it happened in Hakea drupacea, the leaf is warmed to a less degree (dashed line). The shape of the curve is related to the presence of a boundary layer (in Danish grænselag), where the exchange of energy with the surroundings occurs fastest where the boundary layer is thinnest, i.e. along the leaf margins. This is the reason why hoarfrost first settles along the leaf margin and on hairs protruding through the boundary layer.
Transpiration from leaves occurs partly through epidermal cell walls and partly through stomata able to regulate the aperture. However, transpiration through epidermal cell walls is a fixed value depending on the construction of the cell wall. The outermost layer in epidermal cell walls is called the cuticle proper, and in xerophytes it is nearly always of a lamellar type where layers of cutin alternate with layers of wax. Cutin and wax are both lipids and in Hakea drupacea, the lamellar cuticle is extraordinary thick. The left picture is from an immature leaf. Up to 44 lamellae may be present in mature leaves. The dark plumages are cutins on their way to increase the thickness of the cuticle from the inside. In other plants with few or no lamellae, the cuticle is less diffusion tight.
The left diagram shows the diffusion resistances to be overcome during water transport through the plant until it evaporates from the leaves through stomata and cuticle. Some are fixed resistances such as Rc (cuticle), others are variable such as Rs (stomata). In Fick’s diffusion law, some of the fixed resistances are put as K. Then the equation shows that the control stomata have on the transpiration rate (T) depends on the size of the boundary layer resistance Rg. In wind with a thin boundary layer, the degree of stomatal aperture obtains the greatest influence on the rate of transpiration. Δψ is the concentration gradient.
The stomata of the leaves serve to exchange carbon dioxide, oxygen, and water vapor with the surroundings. Stomata consist of two specialized epidermal cells called guard cells. They can change shape depending on pressure changes within the cell, whereby the aperture can open or close. Stomata are often placed in level with the other epidermal cells, but in Hakea and many other xerophytes, they are sunken. This position prolongs the diffusion route for water molecules before they are outside the boundary layer, and hence transpiration rate is reduced. The diagram shows the position of thick stiff walls in the guard cells and thin resilient cell walls, which stretches when the pressure rises, and the aperture opens. The two photos of Hakea drupacea show how deeply sunken the guard cells are. The handmade section above is coloured red (Sudan IV) due to lipids and illustrates how extensive the cutinization of the epidermal outer cell walls is.
Hakea drupacea and probably all other species in the genus are able to permanently block and put stomata out of function and thereby reduce transpiration. It happens as the leaves grow older and as photosynthesis becomes less effective and therefore fewer stomata can supply sufficient CO2. As the leaf gets older, some palisade cells around a stoma will re-differentiate. The otherwise mature palisade cells, which have photosynthesis as their primary function, begin growing and divide one to four times, so that they gradually fill in the substomatal cavity and at last block the stomatal aperture permanently. The re-differentiated palisade cells are called tylosoids. My investigation showed, in the 4th leaf generation 6% of the stomata were blocked, in 6th generation 12%, and in 7th generation up to 50%. The leaves are lost in the 8th generation. The red-brown colour reaction in the cells are due to tannins stored in the central vacuole. Blocking of older stomata happens in many other xerophytes, but it happens by other methods such as blocking from the outside by wax, closing of the aperture when guard cells lose their ability to change form or by suberization of guard cells.
Plenty of supporting mechanical tissue (sclerenchyma and collenchyma) is an important xeromorphic character and Hakea drupacea are well equipped. Mechanical tissue is thick-walled cells and sclerenchyma has lignified walls, i.e. lignin is embedded between the cellulose microfibrils. Sclerenchyma cells can be several mm long and are then called fibres. On the leaf cross section (left), the lignified cell walls light up in the fluorescence microscope and the fibres appear more or less as rounded cells placed around the vascular bundles. The aquifer vessels and tracheids also have lignified cell walls, and hence these cells have a double function.
Other supportive cells are strongly branched and called sclereids. In Hakea they are column shaped with branched endings. They are placed between the palisade cells. The supportive tissue prevents the leaf in collapsing during water stress. If that happens, both photosynthesis and the transport of water and nutrients will more or less stall.
Hakea’s thick-walled sclereids are only moderately lignified and the branches only weakly lignified, hence there is still vacant space for water transport in the cell wall. The two photos above from a light- and a phase-contrast microscope (right) show the sclereid branches lie clinched up against tracheids in the vascular bundle (ring-shaped wall thickenings). Thereby the sclereids get an additional function as prolonged arms of the vascular bundle, and their importance for supplying the palisade cells with water is illustrated by the experiment at left, where a twig has absorbed the fluorescent berberine sulphate.
In many xerophytes, a dense indumentum of dead air-filled hairs covers the leaves. The hairs (trichomes) reflect radiation and thereby reduce leaf temperature, and they increase the thickness of the boundary layer, which reduces transpiration. In Hakea drupacea, T-shaped hairs cover young immature leaves but these hairs are alive with an extremely thin cuticle, so they increase transpiration. This seems to contradict being a xerophyte. However, to Hakea it is important that young growing leaves reach maturity as fast as possible. The T-shaped hairs develop before the stomata are mature and able to drive the transpiration stream. The T-hairs take care of that and secure sufficient water, and nutrients reach the growing leaf. When the stomata become functional and able to control transpiration, the T-hairs become harmful and they are put out of action when the outermost long cell is discarded and only the stalk cell remains.
Fig. A shows a young live T-hair where the outer wall of the stalk cell is cutinized (black). Fig. B shows the long head cell is collapsed due to suberization of the inner wall of the stalk cell (grey) and therefore the pit fields between stalk- and head cell are closed. Finally, the head cell dies and loosens from the stalk cell. D shows how densely the T-trichomes cover the growing leaf. E shows two dead stalk cells. F shows the surface of a T-hair.
In the uppermost right Fig., a young leaf has received a drop of the stain neutral red on its surface and the stain is absorbed in the T-trichomes but nothing has entered the leaf. Therefore, it can be rejected that the hairs should supply the leaves with dew or rainwater. At right, a shoot with growing leaves (light green) is absorbing berberine sulphate. After a few hours, the fluorescent matter is transported with the water to the T-trichomes and this shows how the living trichomes drive the water stream in the young growing leaf.
Hakea drupacea has a few more xeromorphic characters. It concerns water storage tissue, compact mesophyll, and tannins. At right, a diagram shows a so-called mesomorphic leaf of oak (Quercus). Normally, oak does not have problems with water supply during the growth season. The mesophyll between upper and lower epidermis consists of two layers of palisade cells with chloroplasts and a spongy parenchyma with smaller cells and fewer chloroplasts but with a larger volume of air-filled intercellular space. Hakea has two layers of equally long palisade cells but no spongy parenchyma. The central part of the cylindrical leaf consists of more or less isodiametric cells without chloroplasts but with large central vacuoles, which primarily store water to be used by the palisade cells during water stress. There is no intercellular space in the water tissue, since stomata are placed close to the palisade cells, and then there is no need for transporting CO2 through the leaf as in oak. The palisade cells are narrow, and the end walls have a small area for sunlight passing into the cells in contrast to shadow-plants, where the palisade cells are funnel-shaped with a broad end wall. Xerophytes often grow where light intensity is so high, that the chloroplasts need to be shielded to avoid being damaged by overexposure. Accumulation of tannins helps to protect against overexposure. Production of tannins continue through the life of the leaf. The outer palisade layer is filled first and in the 2nd and 3rd growth season tannins are also stored in the inner palisade layer. At last it may be mentioned that palisade cells adjacent to the substomatal cavities in contrast to other cells inside the leaf are provided with an extremely thin cuticle (300-400Å, 1Å = 10-4 µm).
Mammillaria plumosa
Oreocereus sp.
Epostoa lanata
Many xerophytes are covered with dead air-filled hairs. For the plant, it has three advantages. A dense hair-cover increases the thickness of the boundary layer and thereby reduces transpiration, the indumentum reflects part of the sun radiation whereby leaves and stems are warmed lesser, and finally the chloroplasts are better protected from overexposure. In Epostoa lanata, note it is the young growing cells at the shoot tip that is best protected by hairs. In addition, the shown cactus exemplifies that succulence is a xeromorphic character. Succulent plant parts are thick due to the presence of a space consuming water tissue. Both roots, stems, and leaves may be succulent and store water, which the chloroplast containing mesophyll can use during periods of drought.
The shape of the trichomes can be very different between xerophytes. In cacti, they are normally long uni- or multicellular trichomes. In buckthorn (Hippophae rhamnoides), they are formed as shields and called peltate hairs. They consist of a stalk and a multicellular flat head overlapping neighboring peltate hairs, so they create a space with high relative humidity beneath the trichomes and the diffusion resistance is increased to slow down the movement of water molecules to the ambient air.
Rhododendron also has peltate hairs on the lower side of the leaves where the stomata are located but the peltate hairs do not overlap and form a ‘closed’ space as in buckthorn. Nonetheless, they effectively reduce stomatal transpiration, since the stomata are collected in a depression below the shield hairs and there are no stomata in areas between the hairs. It also helps the leaves roll up during drought.
In marram grass (Ammophila arenaria), the leaves are only flat when it rains and the humidity is high, otherwise they are involute with the upper side enclosed. Stomata are only present on the upper leaf side. So-called bulliform cells are located at the bottom of the furrows between the ribs. The pressure in these cells is regulated by pumping water in and out and this will flatten or involute the leaf. Note also the well-developed water storage tissue.
In the heather family (Ericaceae) and Empetraceae, stomata are also hidden in cavities obtained by rolling back or asymmetrical growth of the leaves upper and lower side. Hairs often block the entrance to the cavity. Upper left shows crowberry (Empetrum nigrum), then Cassiope tetragona (flowering at right), and the lowermost is heather (Calluna vulgaris). Their leaves also represent microphylli and the next subject, glandular hairs secreting ethereal oils.
Hymenolepis parviflora (earlier Athanasia parviflora) was mentioned in the beginning of the article as a South-African species with a leaf morphology similar to Hakea drupacea. The young leaves of Hymenolepis has a dense cover of branched trichomes functioning as described for cacti. At right, some of the hairs are removed from a nearly mature leaf so the stomata become visible. Hymenolepis also has secretory cavities (three are visible in the photo at left) and glandular hairs secreting ethereal oils.
The effect of ethereal oils on transpiration is illustrated by the simple experiment shown above at left. Ethereal oils reduce the surface tension of water and force the liquid phase backwards in the capillary tube and similarly in the cavities between the cellulose microfibrills in the cell wall, hence increasing the transpiration resistance. The two photos show the cuticle loosens and is stretched out like a bubble or balloon (asterics) during secretion of the ethereal oils both in the cavities and on the glandular hairs of Hymenolepis. Finally, the cuticle balloon bursts.
The above Fig. illustrates the development of the two types of trichomes in Hymenolepis parviflora from a common mother cell in the epidermis. The upper row shows development of the glandular hairs and the lower row the branched stellate hairs. In both cases, it ends with the stalk cells becoming deeply sunken in the mature leaf, and in both cases, the head cells die. The outermost stalk cell of the glandular hair becomes cutinized and suberized, so that it closes off to the cells of the glandular head, which dies when the ethereal oils are released. The lower stalk cell seems also to have lost importance, while in the branched hairs it undergoes a remarkable development described below.
Above the sunken branched stellate hairs are shown from a mature leaf of Hymenolepis parviflora partly as they look in the light microscope and partly as seen in the electron microscope. The upper right photo is a transversal section of the second outermost stalk cell. The colour photo at left is a paradermal section of a mature leaf showing palisade cells and hair funnels in cross section. There are about 300 funnels per mm2, 30 of which with glandular hairs. There is only one trichome in most funnels, but a few contain two trichomes, that can be of different types. The lower left photo shows a single stellate hair with dead and collapsed branch cells and two live stalk cells.
It is remarkable, that the stalk cells of the stellate hairs are provided with a large number of mitochondria and a particular well-developed labyrinth wall increasing the area of the cell membrane with more than 20 times but at the same time, the cell wall has a relatively thick cuticle. An increased area of the cell membrane normally means that a considerable active transport take place across the membrane, either a secretion or an absorption. Unfortunately, I did not get the possibility to finish the reseach, but since the branches of the stellate hairs fall off on the mature leaf, it is possible that the indumentum has a function similar to that in Hakea drupacea, i.e. to ensure a sufficient transpiration stream with nutrients during leaf growth and to protect the chloroplasts against overexposure. If the lasting stalk cells are water absorbing remains unclear.
Cakile maritima, strandkål
Senecio mandraliscae
Eucalyptus cloeziana
In many xerophytes, a layer of wax called epicuticular wax covers the cuticle, which often gives the leaf surface a bluish tinge. In Senecio above is the wax removed with a finger on a single leaf and then the green colour of the chlorophyll becomes visible. The function of the epicuticular wax is to reflect part of the solar radiation and thereby reduce warming of the leaf and lower the risk of overexposure. Seen with the human eye, the colour the epicuticular wax gives the leaf depends on the form of the wax stumps and the orientation of the wax crystals in the stump as visualized in the Eucalyptus Fig. by Martin & Juniper 1970.
As an example of water absorbing trichomes the Bromeliaceae may be mentioned. The pictures above show Tillandsia usneoides that is an epiphytic xerophyte without roots. It must have all nutrients delivered through the water absorbing peltate hairs on the leaves. At left, the plant is seen in dry and wet condition. In the middle, the densely packed hairs are shown and at right, the dead air-filled head cells are seen. When it rains or dew is falling, water and nutrients are sucked in by capillary forces, and then absorbed by the stalk cell and distributed to the leaf. During water stress, the peltate hairs cover the stomata, increase the diffusion resistance, and reflect sun radiation.
Dasylirion sp.
Psila sp.
Bredemeyera colletioides
Sunken stomata is already mentioned as a xeromorphic character. Above a few more examples are shown. Dasylirion are North American Yucca-alike desert plants, while the two others are South American steppe plants with microphyllous leaves with modest or no contribution to photosynthesis, which the stem has taken over. The stem cortex consists of palisade cells with chloroplasts. Psila is covered by epicuticular wax and like Bredemeyera it has deeply sunken stomata. The black and white photos are from studies by T. W. Böcher and O. Lyshede 1971.
Alluaudia procera
Euphorbia fimbriata
Cereus jamacaru
Leaf orientation may be of importance for reducing transpiration. For example, it is the case in Alluaudia from Madagascar. The small leaves are placed edgeways to reduce incoming radiation and thereby reducing warming and transpiration. In the long dry season, the leaves are discarded and Alluaudia becomes a stem assimilant. Stem succulence with plenty of water storage tissue is often accompanied by microphylli as in Alluaudia and Euphorbia fimbriata. Other Euphorbia species have given up leaves completely just like cactus species. In the subject ‘Rejseindtryk’ other examples are given of microphylli and loss of leaves in the dry season, e.g. Idria and Fouquieria while Ephedra year round are stem assimilants.
A number of plants from warm countries contain two types of chloroplasts where the uncommon type is placed in a cell layer called the bundle sheath surrounding the vascular bundles. These chloroplasts are larger and have fewer stacked lamellae (grana) with chlorophyll. Simultaneously, the bundle sheath cells have thick walls and are isolated from the mesophyll by a suberin lamella (red line in the right Fig.). It has been shown that this kind of leaf anatomy is connected with a special water saving type of photosynthesis called C4, while the normal photosynthesis is called C3. The names come from the first product produced when CO2 is fixed, namely, a carbon compound with 4 and 3 carbon atoms respectively.
Maize (bundle sheath cell with large chloroplasts shown above) is a C4 plant. Its photosynthetic rate continues to increase with higher light intensity in contrast to C3 plants, which reach a maximum where they are light saturated and unable to increase photosynthesis further as shown in the upper right diagram. The diagram also shows a simplified display of normal C3 photosynthesis. The light processes taking place in the grana produce the energy (ATP and NADPH2) to drive CO2 assimilation. The white body is produced starch. It appears that during the respiration processes, CO2 escapes through the stomata, and with increasing light intensity and temperature the CO2 assimilation is equalized and the curve (photosynthetic rate) flattens.
In the lowermost diagram, the course of photosynthesis in a C4 plant is added. The essential difference is that the CO2 produced by respiration does not escape to the ambient air but is exported to the cells outside the bundle sheath and re-assimilated. That is why photosynthesis can continue to increase with higher light intensity but it also means a steeper concentration gradient for CO2 from the air into the leaf. This allows the opening rate of the stomata to be reduced. That means greater resistance for the stomatal transpiration and thereby C4 photosynthesis is water saving.
There are about 8.100 known C4 plants. The majority is monocotyledonous herbs and very few are trees (Paulownia is an example). In Denmark there is only one naturally occurring C4 plant. It is saltwort, Salsola kali growing on the beach or at the foot of dunes. These are localities, which often experiences the highest light intensities and temperatures. The leaves are succulent with a single layer of palisade cells adjacent to a bundle sheath, although, not complete but disrupted by water storage tissue, which in several places reaches the epidermis.
Sporobolus rigens is a C4 grass endemic to Argentine and Chile. It is another example of a disrupted bundle sheath. In the picture, it is the dark stained cells lying against the compact assimilation tissue. Of other xeromorphic characters the central water storage tissue is noted and the supportive fibres around the vascular bundles continue in a bundle sheath extension towards the epidermis. – In the deserts of the Middle East, a few members of Chenopodiaceae are found able to perform C4 photosynthesis without having a bundle sheath, but two necessary enzyme systems are kept separated in the cytoplasm and the central vacuole respectively.
There is one more deviating photosynthetic system, which is a physiological adaptation to being a xerophyte, which is CAM-photosynthesis. The name refers to the system first being observed in the family Crassulaceae (Crassulacean Acid Metabolism), but it is now known from many other families such as Orchidaceae, Cactaceae, and Bromeliaceae but almost exclusively in species with extensive water storage tissue. The method is based on the fact that CO2 initially is fixed in oxaloacetic acid by help of the enzyme PEP (phosphoenolpyruvate) and then stored in malic acid in the central vacuole. This happens during the night, when humidity is relative high and transpiration therefore is modest even if the stomata are open. During the day, sunlight accelerates the photosynthetic light processes and the stored CO2 is released and assimilated through a normal C3-photosyntesis. This occurs with closed stomata and therefore water is saved. The plant at right is Crassula arta from South Africa and Namibia.
Short summary of xeromorphic characters
Large absorbing root surface, proteoid roots – shoot/root ratio small.
Microphylli – ericoid and involute or revolute leaves – stem assimilation.
Heavily cutinized epidermis, lamellar cuticle proper reduces cuticular transpiration.
Plenty of epicuticular wax reflects radiation and reduces warming of the leaves.
Indumentum with dead air-filled trichomes increases the boundary layer and reduces warming.
Water absorbing trichomes for absorbing dew and rain.
Ethereal oils in glandular trichomes and cavities reduce surface tension of water and force the liquid phase of water backwards in the cell wall.
Sunken stomata – many stomata/area – permanent closure of superfluous stomata.
Stoma in furrows or cavities reduce transpiration.
Water storage tissue in cortex, marrow, hypoderm, mesophyll (tannin in vacuoles).
Well-developed conductive tissue and mechanical tissue (sclerenchyma), bundle sheath extensions.
Compact assimilation tissue and reduced intercellular airspace minimize area of exposed cell walls.
C4-photynthesis and CAM-photosynthesis.
Some plants endure dehydration better than others, mosses and lichens in particular.
Annual plants cope with the dry season as embryos surrounded be a protective seed coat.
Glossary (listed according to the order of diagrams):
Bladtemperatur – leaf temperature
Blad – leaf
Luft temp. – air temperature
Grænselag – boundary layer (zone around a plant organ with gradients in RH, CO2 temperature, turbulence)
Vind – wind
Modstande i transpirationsstrømmen – resistances in the transpiration stream
Ledningsstreng – vascular bundle
Rod – root
Jorden – the soil
Æteriske olier i luften – ethereal oils in the air
Fotosyntese – photosynthesis
Lys – light
Lysintensitet – light intensity
Majs – maize (Zea mays)
Sennep – wild mustard (Sinapis arvensis)
Skovsyre – wood-sorrel (Oxalis acetosella)
Glycolsyre – glycolic acid
Oxaleddikesyre – oxaloacetic acid
Æblesyre – malic acid
Strengskedecelle – bundle sheath cell
Kølig nat – cool night
Varm dag – warm day
Grønkorn – chloroplast
Stivelse – starch
Spalteåbning – stoma
Lukkede spalteåbninger – closed stomata
Omvendt glucolyse – reverse glucolysis
H. S. Heide-Jørgensen, March 2021.